Current Work
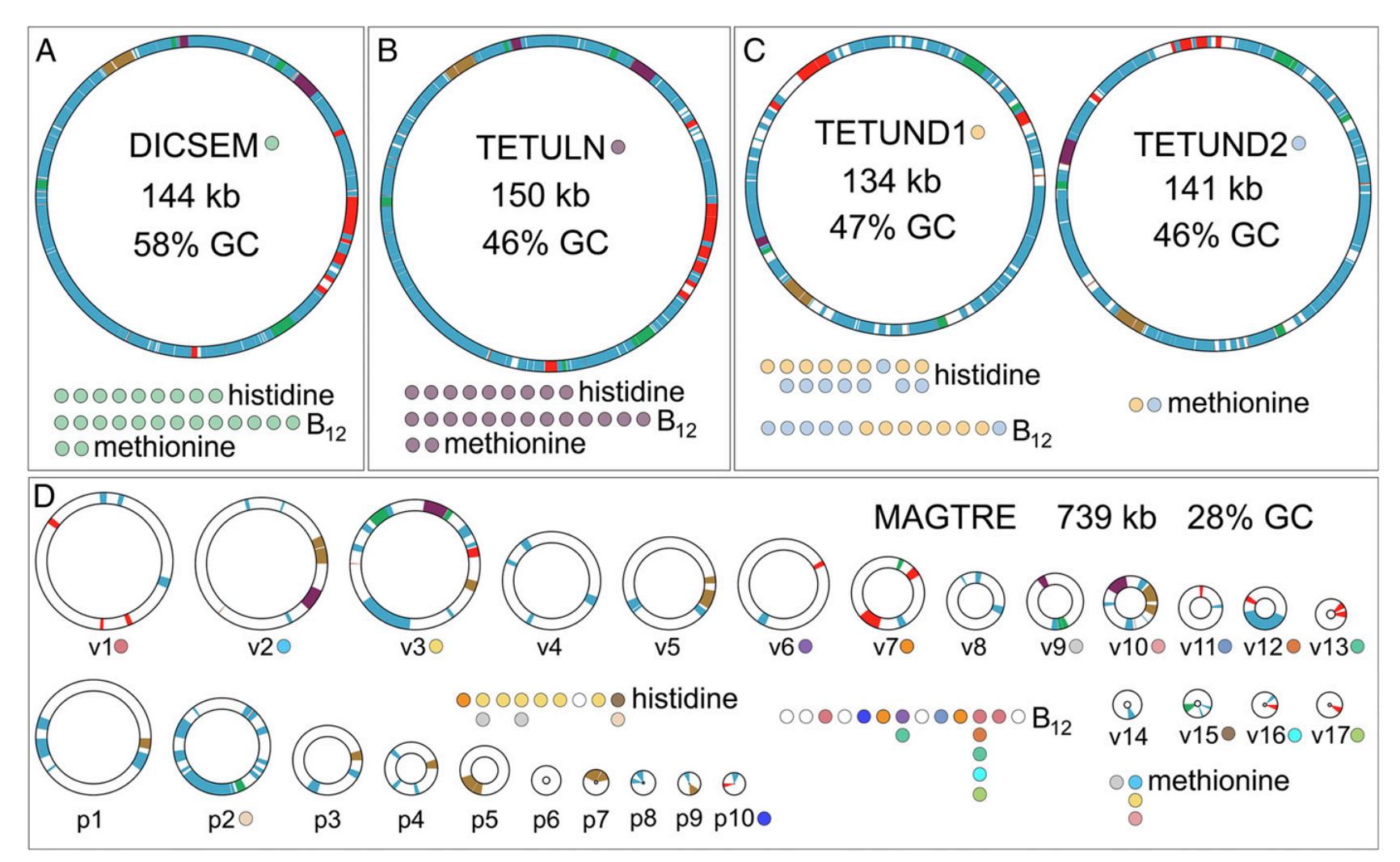
A big focus of our current research is the interactions between insects and microbes and how they function together in a mutually beneficial relationship. Our research uses cicadas as a study system because: a) cicadas have a particularly deficient diet (xylem fluid), b) have longer life cycles than other insects, and c) are diverse and occur worldwide. It turns out that cicadas have particularly strange endosymbiont evolution. Like other plant sucking bugs they have two co-primary bacterial endosymbionts that provide all of their essential amino acids. However, unlike other plant-sucking bugs, one of these endosymbionts exhibits very bizarre evolution. Working with John McCutcheon (U. Montana/ASU) and his students, we have found that this endosymbiont has duplicated its genome to form many copies. All of these copies are passed down to future generations inside the eggs of mother cicadas. Some of the copies are only partially functional. We suspect that this process might be non-adaptive, thus paving the way for other symbionts to take on its role (Van Leuven et al. 2015, Campbell et al. 2015, 2017, 2018, Łukasik et al. 2018).
Following from work by the McCutcheon and Japanese collaborators, we have found that in many cicada species, the bizarrely evolving endosymbionts (but not their partners) have been replaced by a yeast-like fungal symbiont. The interesting thing about the fungal symbionts is that they are derived from a parasitic progenitor. The fungal parasites, (several Ophiocordyceps species) normally attack the underground cicada nymphs and kill them after causing them to dig upwards and die just below the surface. As the cicada dies, masses of fungal hyphae burst out of its head and form a mushroom-like fruiting body on the forest floor (the mushrooms look like pink antlers). We have found that in New Zealand, all of the 50+ endemic cicadas have Ophiocordyceps fungal symbionts replacing the odd-ball primary symbiont (Haji et al. in revision). We hypothesize that they brought the fungus with them when they colonized NZ because the parasitic version of that fungus does not occur in cicadas in NZ (instead their nymphs are sometimes killed by a different fungal parasite, Isaria that looks like little white cauliflowers on the ends of antlers on the forest floor). In collaboration with John McCutcheon and led by my Postdoc Eric Gordon and former McCutcheon-lab postdoc Piotr Łukasik (Jagiellonian U.), we are surveying cicadas worldwide to understand the complexity of both fungal and bacterial endosymbiont evolution. Including North American Neotibicen and relatives led by Honors Scholar Jason Vailionis. We are continuing studies of Patagonian cicadas with collaborator Jeffry Cole (Pasadena Community College), Claudio Veloso (U. Chile), and Pablo Pessacq (Centro de Investigaciones Esquel de Montaña y Estepa Patagónicas).
To study the evolution of cicadas worldwide, we have created a phylogenomic tree in collaboration with Emily and Alan Lemmon of the Laboratory for Anchored Phylogenomics at Florida State University. This tree is based on nuclear, organelle, and symbiont genomes. We have completed various subclades of the tree and soon will have sequences of cicadas from most tribes and genera. This 20-year-long project has been possible through collaboration with many cicada scientists around the world and support from the National Science Foundation, the National Geographic Society, the Fulbright Foundation, the University of Connecticut, and the New Zealand Marsden Fund.
Recently, we discovered that the earliest split in the cicada tree of life showed, unexpectedly, that a tiny, pale-green cicada in the genus Derotettix, living in degraded salt flats of central Argentina is the sister group to all other modern singing cicadas (Simon et al. 2019). Although Derotettix was described in 1882, no one had noticed that it was particularly unusual until we sequenced its DNA. We estimated that the first cicada split occurred over 100 million years ago (in the late Cretaceous) when dinosaurs still roamed the earth. Oddly, there are only two species of Derotettix, both in central Argentina, that survived to represent their 100-year-old lineage. We hypothesize that they survived because they were able to adapt to dry, salty habitats that were created by multiple marine inundations (epicontinental seas) in central Argentina, the last and greatest of which was in the mid Miocene 13-15 million years ago. Our Family Cicadidae phylogenomics work is progressing in collaboration with Chris Owen (Smithsonian-USDA), led by Postdoc Eric Gordon and PhD student Mark Stukel.
We began our biogeographic studies with the largest tribe of cicadas, Cicadettini (>1000 species worldwide) led by Postdoc David Marshall. Using molecular phylogenetics, we found that this tribe originated and diversified in Australia and later spread to the rest of the world (Marshall et al. 2016). PhD student Chris Owen discovered that diversity in the largest Australian genus is much greater than previously understood (he has created five new genera and described 21 new species) (Owen et al. 2015, 2017, Owen and Moulds 2016). In Australia, we are interested in the effect of increasing aridification on diversification over the last 30 Myr. Results from this grant formed the basis for an NSF award to study the biogeography of all cicada tribes worldwide (more than 3000 species), led by Research Scientist David Marshall and Technician Kathy Hill for phylogenetics and now Mark Stukel and Eric Gordon for phylogenomics with continued collaboration with David Marshall (UCONN), Max Moulds (Australian Museum) and former Simon Lab postdoc, Ben Price (The Natural History Museum, London). We have additional cicada collaborators in more than a dozen countries worldwide. Our biogeographic studies are based on a molecular phylogeny constructed from mitochondrial, nuclear and endosymbiont DNA data and will incorporate information on continental movements and climate change.
New Zealand’s well-studied and dramatic landscape and climate changes make this system ideal for exploring the impact of physical changes on speciation. We have now studied the molecular evolution, natural history, courtship songs, and ecology of every one of the 60 taxa of NZ cicadas (Arensburger et al. 2004a; Buckley et al. 2001a, b, c, 2006; Buckley and Simon 2007; Marshall et al. 2008, 2009, 2011, Simon et al. 2009; Hill et al. 2009, Ellis et al. 2015, Banker et al. 2017, Bator et al. in press). We traced the origin of NZ genera to Australia (Buckley et al. 2002; Arensburger et al. 2004b) and discovered that all of the diversity we see today in NZ arose in the last 10-15 Ma. Two pulses of diversification occurred in association with mountain building followed by more-or-less steady diversification throughout the ice ages (ca. 2 Myr). Based on courtship song, combined with DNA and morphological evidence, we estimate time to speciation at ca. one to two million years. Entering a new phase of NZ cicada evolution, led by PhD student Mark Stukel with participation by research undergrad Alex Porczak, we are exploring phylogenomic relationships and the effect of nucleotide bias, outlier genes, number of genes, incorporation of organelle data, and hybridization on the reconstruction of gene trees and species trees. We continue to collaborate with former Simon Lab PhD student, Thomas Buckley, now at Landcare Research, Auckland, NZ.
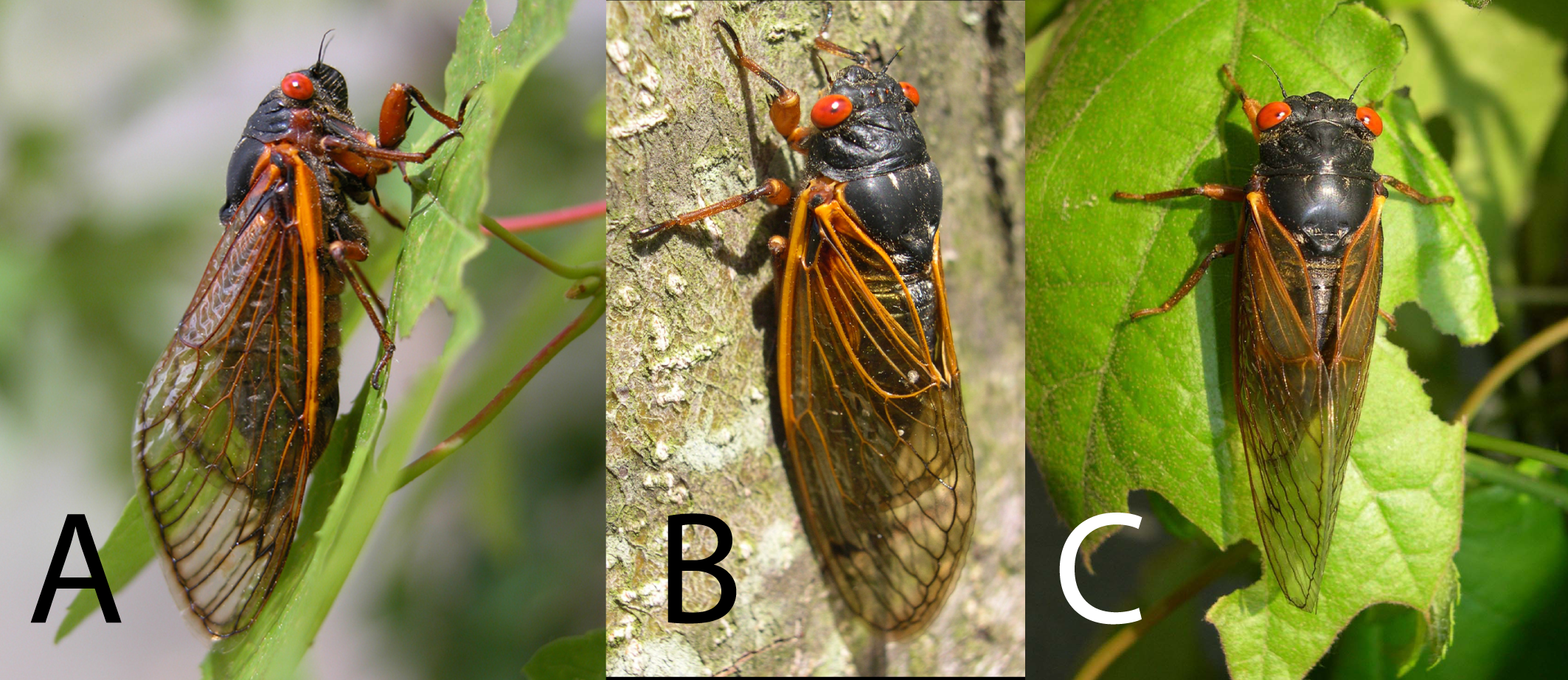
Species are not guaranteed eternal existence. Like climate or habitat change, hybridization can lead to extinction. As well as climate or habitat change hybridization also can lead to extinction. Our research is demonstrating that animal species exhibit far more hybridization than previously realized. We have an NSF proposal under development to study interactions at hybrid zones between more than 20 NZ cicada species pairs with divergence times ranging from 20,000 to 7 million years. We predict that while the ability to hybridize decreases with increasing genetic distance, success is highly dependent on behavioral differences. PhD student, Beth Wade, and proposed postdoctoral researcher, Lindsay Popple, will study the contact zones. Our phylogeographic studies to date (Marshall et al. 2008, 2009, 2011) provide critical background data to estimate relationships and divergence times. We will estimate gene flow across contact zones and study the extent of introgression over geography. Some of the species complexes may turn out instead to be “hybrid swarms”. Much of the suspected introgression may be associated with habitat modification by humans. A parallel project is underway to study the phylogeography and evolution of the NZ genus Maoricicada (Buckley et al. 2001, Hill et al. 2009, Hill et al. in prep). An understanding of human-aided spread in the past can help to design future strategies to preserve species diversity.
D. The Documentation of Biological Diversity.
As part of a NSF effort to address the critical need for taxonomic expertise to identify our disappearing biological diversity, we are participating in an NSF PEET project (Partnerships to Enhance Expertise in Taxonomy). Along with colleagues from the NY State Museum and the Illinois Natural History Survey, we have been training new experts in the taxonomy of our group, Auchenorrhynchan Hemiptera (ca. 50,000 described, and ca. 250,000 undescribed). This work will be followed by an NSF submission in June to focus on Auchenorrhyncha in the Philippines, a relatively unexplored hot spot of insect biodiversity. Ph.D. students Geert Goemans and Chris Owen, and postdoctoral researcher, Young June Lee, are the primary researchers focusing on taxonomy. Kathy Hill and David Marshall are focusing on deeper level taxonomy of the family in addition to their studies of biogeography and phylogeography mentioned above. They also study the taxonomy of NZ cicadas and various Australian and North American genera (especially Gudanga and Tibicen). John Cooley is studying the systematics of the genus Okanagana and relatives.
The first DNA I sequenced was a section of the mitochondrial small subunit ribosomal RNA gene (12S rRNA). Studying this and related segments, deciphering their secondary structure and comparing their evolution to that of the homologous segment in other species (Simon et al. 1990, 1994, 1996; Hickson et al. 1996, Hickson et al. 2000, Buckley et al. 2000, Saux et al. 2003, Carapelli et al. 2004) led to a great appreciation for the strong variation in rate and pattern of DNA evolution among nucleotide sites and across lineages, and how this rate variation influences phylogenetic tree construction. We showed that incorporating information on secondary structure is critical for alignment of rRNA, that variation in rate and pattern of DNA evolution is strong and that ignoring this variation has more of an impact on phylogenetic analysis and molecular-clock dating than any other factor (Simon et al. 1994, 1996; Frati et al. 1997; Sullivan et al. 1995, 1996; Gogarten et al. 1996; Buckley et al. 2001, 2002; Marshall et al. 2006; Simon et al. 2006). Our work also addressed problems of experimental design/sampling (Simon and Archie 1985, Archie et al. 1989), molecular methodology (Simon et al. 1993, Simon et al. 1994, Phillips and Simon 1995, Simon et al. 2006), phylogenetic methodology (Buckley et al. 2001, Buckley et al. 2002, Buckley et al. 2006), and data partitioning (Marshall et al. 2006).
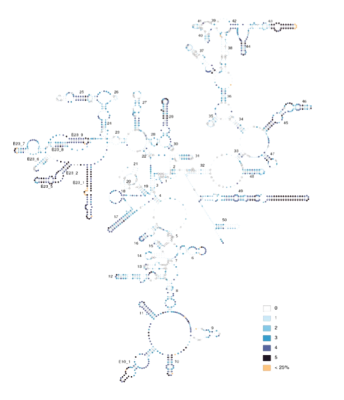
You can read more about some former research projects here.